Abstracts
Chemotherapy is the only validated therapy for the treatment for the neglected diseases leishmaniasis and malaria. However, the emergence of drug resistance, collateral effects and long-term treatment encourage the development of new and more efficient drugs. The Amazon tropical forest includes the richest areas of biodiversity in the world, including a great number of microbes, plant and animal species that produce a source of interesting biologically active molecules. Several of these molecules, obtained from plant extracts and frog venom have leishmanicidal and plasmodicidal activity, highlighting the potential of this biodiversity for the development of new drugs. In research, modern approaches in new drug development are carried out using combinatorial chemistry, high-throughput screening, bioinformatics, molecular interaction, crystallography and dynamic studies of cellular and systemic toxicity. In Brazil, these techniques are mainly present in only a few academic groups with no efficient connection to industry. The problem associated with over-regulation for accessing the biological material in restricted areas, local populations and indigenous areas places major barriers in the path of research and development of new drugs. Thus, the association of academic research groups in Brazil, encouraged and supported by government and industry, is essential to overcome these major barriers related to the development of new products for treatment of neglected diseases from Amazonian biodiversity in future years.
Amazonia; biodiversity; leishmaniasis; Leishmania; malaria; Plasmodium; drug development
A quimioterapia é o único procedimento farmacológico validado para a terapêutica da leishmaniose e da malária, consideradas doenças negligenciadas para o desenvolvimento de fármacos pelas indústrias farmacêuticas. Com a não renovação medicamentosa, o surgimento de resistência, os efeitos colaterais e o longo período de tratamento indicam a necessidade do desenvolvimento de novos e mais eficientes fármacos. A Floresta Amazônica é a região com a maior biodiversidade do planeta, com uma riqueza de animais e plantas produtoras de moléculas com atividades biológicas relevantes para o desenvolvimento e exploração biotecnológica. Algumas destas moléculas, obtidas de extratos vegetais e de venenos de anuros, apresentam atividade leishmanicida e plasmodicida, o que demonstra o potencial desta biodiversidade para a investigação de novas drogas. A moderna abordagem na pesquisa de novos fármacos envolve a associação de química combinatória, high-throughput screening, bioinformática, interação molecular, cristalografia e o estudo dinâmico de toxicidade sistêmica e celular, que atualmente no Brasil, estão distribuídas em poucos grupos acadêmicos sem a devida associação industrial. Esta deficiência, agregada ao excesso de regulamentação para o acesso ao material biológico, sobretudo, proveniente de unidades de conservação, populações tradicionais e nações indígenas, é um importante entrave para o desenvolvimento deste tipo de pesquisa. A associação de grupos de pesquisa do Brasil, estimulados por políticas governamentais de financiamento acadêmico e industrial, são essenciais para a superação destas dificuldades, de forma que nos próximos anos possam surgir novos produtos para terapia de doenças negligenciadas oriundas da biodiversidade amazônica.
REVIEW
Amazonian biodiversity: a view of drug development for Leishmaniasis and malaria
Leonardo de Azevedo CalderonI, II; Izaltina Silva-JardimII; Juliana Pavan ZulianiII; Alexandre de Almeida e SilvaII, III; Pietro CiancagliniIV; Luiz Hildebrando Pereira da SilvaII; Rodrigo Guerino StábeliI, II,* * e-mail: stabeli@unir.br
ICentro de Estudos de Biomoléculas Aplicadas a Medicina Prof. Dr. José Roberto Giglio, Núcleo de Saúde, Universidade Federal de Rondônia, BR 364 Km 9.5, 76800-000 Porto Velho-RO, Brazil
IIInstituto de Pesquisas em Patologias Tropicais de Rondônia, Rua da Beira 7671, BR 364 km 9.5, 76812-245 Porto Velho-RO, Brazil
IIILaboratório de Bioecologia de Insetos, Departamento de Biologia, Núcleo de Ciência e Tecnologia, Universidade Federal de Rondônia BR 364 Km 9.5, 76800-000 Porto Velho-RO, Brazil
IVDepartamento de Química, Faculdade de Filosofia Ciências e Letras de Ribeirão Preto, Universidade de São Paulo, 14040-901 São Paulo-SP, Brazil
ABSTRACT
Chemotherapy is the only validated therapy for the treatment for the neglected diseases leishmaniasis and malaria. However, the emergence of drug resistance, collateral effects and long-term treatment encourage the development of new and more efficient drugs. The Amazon tropical forest includes the richest areas of biodiversity in the world, including a great number of microbes, plant and animal species that produce a source of interesting biologically active molecules. Several of these molecules, obtained from plant extracts and frog venom have leishmanicidal and plasmodicidal activity, highlighting the potential of this biodiversity for the development of new drugs. In research, modern approaches in new drug development are carried out using combinatorial chemistry, high-throughput screening, bioinformatics, molecular interaction, crystallography and dynamic studies of cellular and systemic toxicity. In Brazil, these techniques are mainly present in only a few academic groups with no efficient connection to industry. The problem associated with over-regulation for accessing the biological material in restricted areas, local populations and indigenous areas places major barriers in the path of research and development of new drugs. Thus, the association of academic research groups in Brazil, encouraged and supported by government and industry, is essential to overcome these major barriers related to the development of new products for treatment of neglected diseases from Amazonian biodiversity in future years.
Keywords: Amazonia, biodiversity, leishmaniasis, Leishmania, malaria, Plasmodium, drug development
RESUMO
A quimioterapia é o único procedimento farmacológico validado para a terapêutica da leishmaniose e da malária, consideradas doenças negligenciadas para o desenvolvimento de fármacos pelas indústrias farmacêuticas. Com a não renovação medicamentosa, o surgimento de resistência, os efeitos colaterais e o longo período de tratamento indicam a necessidade do desenvolvimento de novos e mais eficientes fármacos. A Floresta Amazônica é a região com a maior biodiversidade do planeta, com uma riqueza de animais e plantas produtoras de moléculas com atividades biológicas relevantes para o desenvolvimento e exploração biotecnológica. Algumas destas moléculas, obtidas de extratos vegetais e de venenos de anuros, apresentam atividade leishmanicida e plasmodicida, o que demonstra o potencial desta biodiversidade para a investigação de novas drogas. A moderna abordagem na pesquisa de novos fármacos envolve a associação de química combinatória, high-throughput screening, bioinformática, interação molecular, cristalografia e o estudo dinâmico de toxicidade sistêmica e celular, que atualmente no Brasil, estão distribuídas em poucos grupos acadêmicos sem a devida associação industrial. Esta deficiência, agregada ao excesso de regulamentação para o acesso ao material biológico, sobretudo, proveniente de unidades de conservação, populações tradicionais e nações indígenas, é um importante entrave para o desenvolvimento deste tipo de pesquisa. A associação de grupos de pesquisa do Brasil, estimulados por políticas governamentais de financiamento acadêmico e industrial, são essenciais para a superação destas dificuldades, de forma que nos próximos anos possam surgir novos produtos para terapia de doenças negligenciadas oriundas da biodiversidade amazônica.
1. Amazonian Biodiversity
Biological diversity is not evenly distributed across the planet. Tropical rainforests of the world are the most species-rich biome, and probably include more than half of the number of species on earth. Approximately 70% of the world's species are distributed in only 12 countries: Australia, Brazil, China, Colombia, Ecuador, India, Indonesia, Madagascar, Mexico, Peru, and Zaire.1-3 Tropical forests in the Americas are consistently more species rich than those in Africa and Asia.4 As the largest tract of tropical rainforest in the Americas, the Amazon tropical forest has unparalleled biodiversity richness, comprising the richest biodiversity area in the world, including a great number of microbes and plant and animal species.4-9 Frequently, new species are added to a truly huge diversity list. Recent compilations indicate at least 40,000 plant species, 427 mammals, 1294 birds, 378 reptiles, 427 amphibians, and around 3,000 fishes have been scientifically classified in the region.10
It is believed that one third of the world's species inhabit the Amazon tropical rainforest, however one of the great gaps in Amazonian knowledge is the cataloguing and taxonomy of plants.11 Actually it is estimated that the distribution of unknown flora in the Amazon has long been underestimated and that plant biodiversity probably includes at least 3 times more plant species in the Amazon than are currently known.11
1.1 Biodiversity and drug development
Plants offer a vast source of molecules that present different effects in human homeostasis.12 These molecules, called natural products, have been extensively recognized as an important source of most of the active components in therapeutically effective medicines. One of the reasons for this success is, most of the time, natural products are more readily absorbed than synthetic drugs.13 Before the advent of high-throughput screening and post-genomic technologies more than 80% of drug substances were natural products or inspired by a natural compound.14 This fact illustrates the importance of natural products for human health.
Natural products are not always used just as medical products but their actions has also helped reveal important aspect of physiology by interactions with membrane channels, receptors and metabolic pathways.13-16
Comparising information available on sources of new drugs from 1981 to 200717,18 has indicated that almost half of the drugs approved since 1994 are based on natural products.13 Between the years of 1981 to 2006, the U. S. Food and Drug Administration (FDA) has approved 1184 new drugs among wich 609 (51.4%) were natural-products-related: 55 of them were natural products, 270 derived from a natural product by semisynthetic modification, 52 made by total synthesis were the pharmacophore came from a natural product, and 232 were sythetized mimicking a natural product.19 Comparisons of the information presented on sources of new drugs from 1981 to 200718,19 indicate that almost half of the drugs approved since 1994 are based on natural products. Thirteen natural-product-related drugs were approved from 2005 to 200718 and, as pointed out by Butler,18 five of these represented the first members of new classes of drugs: the peptides exenatide and ziconotide, and the small molecules ixabepilone, retapamulin and trabectedin.13
Despite the advantages of natural products and past successes, many large pharmaceutical companies have scaled back the use of natural products in drug discovery screening.13 This has been because of the noted disadvantages of their use that include the difficulties in sourcing and supply, complexities of natural product chemistry and the inherent delay of working with them, together with complications connected to legal regulation involving intellectual property rights, access to genetic resources, traditional knowledge and related benefit sharing.
Complications involving biodiversity's legal access regulations in Brazil today comprise the greatest problem for the scientific and technological development of the national pharmaceutical industry in the country with the greatest biodiversity in the world.
According to Harvey,13 although this is a time when pharmaceutical companies cut back on their use of natural products in drug discovery, there are many promising drug candidates in the current development pipeline that are of natural origin. Technical drawbacks associated with natural products research have been lessened, and there are better opportunities to explore the biological activity of previously inaccessible sources of natural products. With the increasing acceptance that the chemical diversity of natural products it is essencial to provide core support for the development of new drugs so that in the future there will be further expansion in the use of drugs based on natural products, whether in nature or developed from chemical libraries.
1.2 Traditional medicine and ethnopharmacology
Traditional medicine is a comprehensive term used to refer either to systems such as Chinese medicine, Indian ayurveda and Arabic unani medicine, or to various forms used in indigenous and traditional communities. Traditional medicine is characterized by the use of plants, animals and mineral substances by arcaic communities for therapeutical purposes to treat various diseases.20 Studies of the pharmacological aspects of these medicines by ethnopharmacological approaches reveal several active components derived form plants used in these ancient practices, with several historic examples.
Jesuit priests reported in the seventeenth century that South America Indians used a tea-like drink prepared from the bark of a native tree from Peru21 to treat some types of fever. In 1742, this tree was identified as Cinchona sp22 (Rubiaceae) and in 1820 the alkaloid quinine was purified from the bark of Cinchona.22,23 A tea made from Artemisia annua (Asteraceae) has been used for treat intermittent fevers in China since 168 B.C. In 1972, the active ingredient artemisinin, a sesquiterpene lactone with antimalarial activity, was isolated from this plant. Indians from the north and northeast of Brazil have used oil from Copaifera spp (Fabaceae) since the 19th century to heal wounds and other skin diseases, probably, based on observations of animals rubbing on copaiba's trunks.24
Traditional medicines are widely disseminated and it is estimated that 80% of the world´s population depends on them as a primary health source.25 In Brazil, populations of rural areas and forests rely on popular and traditional medicine for treatment of many infectious diseases. Some species are included in prescriptions for therapeutic purposes such as the healing of wounds, inflammation due to microbial or parasitic infections, skin lesions, and ulcers.12 In some cases, the same plant may be used for different purposes.26 It is the general consensus that traditional medical use of several biological sources indicates the presence of biologically active compounds. Bourdy et al.27 relate their experience over years with different ethnic groups from South America that showed that whenever a strong incidence of malaria is found, then half of the species related as "true" antimalarials had in vitro and in vivo activity. In spite of that, Bourdy et al.27 also related that harvesting season and different preparation protocols in the laboratory lead to different results with in vitro and in vivo models. Possibly, the inactivity or even poor activity reported in several scientific articles worldwide using different biological sources possess biased conclusions.
1.3 Neglected diseases
Leishmania and Plasmodium are protozoan parasites responsible for a spectrum of diseases known as leishmaniasis and malaria. These diseases are classified as neglected diseases due to lack of financial investments by the world pharmaceutical industry and, when associated with HIV co-infection, present a serious public health problem in the majority of the developing countries in the tropical regions of the world.28 Human malaria is an infectious disease caused by one or more species of Plasmodium (i.e., P. falciparum, P. vivax, P. ovale, and P. malariae). Malaria remains a devastating global problem with 350-500 million cases reported and an estimates one million deaths annually, 80% of them in sub-Saharan Africa.29 Forty-nine percent of the world's population lives in risk areas (e.g., 109 countries in Africa, Asia, the Middle East, Eastern Europe, Central America and South America, the Caribbean, and Oceania).29 Another tropical disease with relevant importance regarding public health is leishmaniasis, a broad spectrum of parasitic diseases caused by obligate intracellular protozoa.30-33 Leishmaniasis comprises two major forms: cutaneous, which causes skin sores, and visceral, which affects internal organs of the body (e.g., spleen, liver, and bone marrow). It is estimated that 350 million people in approximately 90 countries around the world are exposed to infection by Leishmania and that some 1.5-2 million people are infected annually.34 In the Old World, leishmaniasis was common in Asia, the Middle East, Africa (particularly East and North Africa, with some cases elsewhere), Southern European regions, but not in Australia or the South Pacific. In the Americas, leishmaniasis occurs from Northern Mexico (seldom in the Southern United States) to Northern Argentina; but not in Chile, Uruguay and Canada. More than 90% of the world's cases of cutaneous leishmaniasis are in Afghanistan, Algeria, Brazil, Iran, Iraq, Peru, Saudi Arabia and Syria, and 90% of the world's cases of visceral leishmaniasis occur in Bangladesh, Brazil, India, Nepal and Sudan.34
1.4 Malaria and leishmaniasis validated therapies
The first choice treatments for these diseases are based on a limited number of chemotherapeutic agents characterized by high toxicity and cost, which have made treatment inefficient since the 1980's in many areas of high-transmission.35Quinine from Cinchona sp22 (Rubiaceae) was the drug most used to treat malaria until the First World War (1914-1918). During this conflict, new molecules with antimalarial activity were synthesized, such as 9-aminoacrinidine (quinacrine, mepacrine) which was synthesized in the 1920's and commercialized in 1930 under the name of atabrine.36 In 1944, quinine was synthesized in the laboratory,22 encouraging the development of several drugs, among them the amodiaquine, primaquine and chloroquine. These drugs are generally used to treat malaria due to their low cost, tolerance, safety in the treatment of pregnant women, lack of toxic effect in recommended doses, and high effectiveness in curing the disease.37,38 Chloroquine-resistant P. falciparum, first detected in 1961 in Vietnam, was reported in several American soldiers,39 motivating an intensive program of research for antimalarial new agents. Dapsone and pyrimethamine/sulfadoxine were introduced in prophylaxis.21,40 Pyrimethamine was also sold in combination with sulfalene and with dapsone.41 Later on, two other highly effective compounds against strains resistant to P. falciparum were approved by the FDA: mefloquine42 and halofantrine.38,43 However, there are reports of resistance to both compounds.44-46 Pentavalent antimonials have been recommended for the treatment of leishmaniasis for over 50 years.47 Unfortunately, the treatment with these drugs leads to well-described adverse reactions, and parasite resistance to these drugs is increasing in some areas, where limited or no efficacy is observed.48,49 Diamidine pentamidine and amphotericin B are used as second choices for leishmaniasis treatment. However, the use of these drugs is limited due to their toxicity or unconventional administration.50 Newer drugs, such as the lipid formulations of amphotericin B, have been effective in the treatment of visceral leishmaniasis.47,51,52 Unfortunately, the prohibitive cost of the new formulations of this drug inhibit its use by the majority of patients with visceral leishmaniasis.51
Drug intervention is the only mechanism of malaria and leishmaniasis treatment approved by the World Health Organization. However, the chronic drug resistance resulting from the recommended therapy, associated with the collateral effects, has stimulated the search for alternative treatments.30,31 In addition to this long-term therapy associated with toxic effects for both diseases frequently leads to patients abandoning treatment, indicating the need for new drug searches.
1.5 Natural products from Amazonian biodiversity and their potential in chemoterapy of neglected diseases
Among natural products, the alkaloids comprise the largest single class of secondary plant metabolites. They play an important role in plant defense against a variety of microorganisms and animals, having a remarkable range of pharmacological activity. 19,53-57 These compounds present an enormous structural diversity. Currently, several alkaloids58,59 and other isolated molecules55,60-62 have biological activity against important parasites including Leishmania sp and Plasmodium sp. These parasites are the etiologic agents of the most important neglected diseases in the world. These molecules are promising candidates in the development of antiparasitic drugs and as tools for the elucidation of important metabolic pathways of these parasites. Extracts and purified secondary metabolites obtained from plants belonging to the genus Abuta (Menispermaceae),65Aspidosperma (Apocynaceae),62Bidens (Asteraceae),63Cinchona (Rubiaceae),64Croton (Euphorbiaceae),65Erythrina (Fabaceae),66 Picrolemma (Simaroubaceae),62Piper (Piperaceae),67 Pothomorphe (Piperaceae),62,68,69Quassia (Simaroubaceae),70-72Remijia (Rubiaceae),64Simaba (Simaroubaceae),72,73Tabebuia (Bignoniaceae),74Xylopia (Annonaceae),65 and Zanthoxylum (Rutaceae),75 found in Amazonia, presented effective antimalarial activity. Some of the most potent molecules against Plasmodium falciparum were obtained from the bark and roots of Amazonian species belonging to these genera: neosergeolide, a terpenoid quassinoid from Picrolemma sprucei (Simaroubaceae);62 simalikalactone D, a terpenoid quassinoid from Quassia amara (Simaroubaceae)71 and Simaba orinocensis (Simaroubaceae);72 aspidocarpine, an alkaloid from Aspidosperma desmanthum (Apocynaceae);62 orinocinolide, a terpenoid quassinoid from Simaba orinocensis (Simaroubaceae);72 and ellipticine, an alkaloid from Aspidosperma vargasii (Apocynaceae).62 These molecules present potent antimalarial activity against chloroquine-resistant Plasmodium falciparum in vitro (IC50= 1.0, 3.7, 7.0, 8.5, and 18.0 ng mL-1 respectively). Other purified molecules and extracts from the genera Annona (Annonaceae),76Baccharis (Asteraceae),26Calophyllum (Clusiaceae),77Cassia (Fabaceae),78Copaifera (Fabaceae),79 Croton (Euphorbiaceae),80Guatteria (Annonaceae),81 Jacaranda (Bignoniaceae),82Lippia (Verbenaceae),26Piper (Piperaceae),83Plectranthus (Lamiaceae),26Stachytarpheta (Verbenaceae),84 and Pourouma (Moraceae),85 that also have Amazonian species, present effective antileishmanial activity.
Secondary metabolites isolated from Annonaceae plants had leishmanicidal activity against different species of Leishmania. Berberine, a quaternary isoquinolinic alkaloid found in a number of plant families (Annonaceae, Berberidaceae, Menispermaceae), is one of the alkaloids with the highest leishmanicidal activity. This metabolite is the main constituent in various folk remedies used in the treatment of cutaneous leishmaniasis, malaria and amoebiasis.86 Berberine has been used clinically for the treatment of leishmaniasis and it has been demonstrated that it possesses significant activity both in vitro and in vivo against several species of Leishmania. At a concentration of 10 g µL-1 it effectively eliminates L. major parasites in peritoneal mice macrophages.86, 109,110
Species from the Annonaceae family have had their alkaloids isolated and investigated for their leishmanicidal activities. The isolated compound anonaine, an isoquinoline alkaloid from the roots of Annona spinescens (Annonaceae), produces total lysis of promastigote forms of L. amazonensis, L. braziliensis and L. donovani with 25, 50, and 100 µg mL-1 doses respectively.89 Liriodenine, an isoquinoline alkaloid from the roots and bark of Annona spinescens and Annona foetida (Annonaceae) exhibited antileishmanial activity in vitro against promastigotes of L. braziliensis (IC50= 34.8 µmol L-1).76 Isoguattouregidine, an isoquinolinic alkaloid isolated from the bark of Guatteria foliosa (Annonaceae), causes a total lysis of the parasites of L. donovani and L. amazonensis when evaluated at a concentration of 100 µmol L-1.89,112
The alkaloids xylopine and nornuciferine from Guatteria amplifolia (Annonaceae), and cryptodorine, nornantenine, isodomesticine, orisodomesticine, nantenine, and neolitsine from Guatteria dumetorum (Annonaceae) demonstrated significant activity against Leishmania mexicana. Xylopine was among the most active compounds (LD50 = 3 µmol L-1) and showed a 37-fold higher toxicity towards L. mexicana than macrophages.90 Neolitsine effectively reduced the parasite growth at a concentration of 15 µmol L-1.81
Rosa et al.80 investigated the leishmanicidal activity of the essential oil rich in the terpenic alcohol linalool extracted from Croton cajucara (Euphorbiaceae) against Leishmania amazonensis. The LD50 values of C. cajucara essential oil and purified linalool on the viability of L. amazonensis promastigotes were 8.3 ng mL-1 for essential oil and 4.3 ng mL-1 for purified linalool, and the LD50 for amastigotes were 22.0 ng mL-1 and 15.5 ng mL-1, respectively. These compounds have a potent leishmanicidal activity due to their disruption of flagellar membranes, mitochondrial swelling, and damages in the organization of the nuclear and kinetoplast chromatins. The essential oils were not toxic to mammalian cells and increased nitric oxide production by mouse peritoneal macrophages.80
Species of the Copaifera genus (Fabaceae) are important medicinal plants of the Amazonian region. They supply an oil, known as copaiba oil or balsam of copaiba, widely commercialized all over Brazil due to its anti-inflammatory and healing agent properties, as has been acknowledged for centuries.91-93 Trypanocidal and leishmanicidal activities of the copaiba oils were mentioned in some ethnopharmacological studies.91,92 Santos et al.24 screened eight different kinds of Brazilian copaiba oils for antileishmanial activity. Oil from Copaifera reticulata had activity against promastigote, axenic amastigote and intracellular amastigote forms of Leishmania amazonensis, with IC50 values of 5, 15, and 20 µg mL-1, respectively.24
Another potential plant family, currently under investigations, that presents important perspectives for drug development is the Piperaceae family. These plants are widely distributed in tropical and subtropical regions of the world, and are often used as food flavouring agents, in traditional medicines, and as pest control agents.94 Phytochemical research carried out with Piper species revealed different classes of natural products such as alkaloids, amides, cyclopentenedione derivatives, dihydrochalcones, essential oils, flavonoids, lignans, neolignans, and phenylpropanoids.94-102 The bioactive products of Piper have several biological activities including anti-inflammatory, antimicrobial, antifungal, insecticidal and cytotoxic effects.103-106 Thirteen benzoic acid derivatives isolated from Piper glabratum (Piperaceae) and P. acutifolium (Piperaceae) were evaluated in vitro against the promastigote forms of Leishmania spp., Trypanosoma cruzi, and Plasmodium falciparum.67 Among the evaluated compounds, methyl 3,4-dihydroxy-5-(3'-methyl-2'-butenyl)benzoate exhibited the leishmanicidal effect (IC50 13.8-18.5 µg mL-1) against the three Leishmania strains used, and methyl 3,4-dihydroxy-5-(2-hydroxy-3-methylbutenyl)benzoate, methyl 4-hydroxy-3-(2-hydroxy-3-methyl-3-butenyl)benzoate, and methyl 3,4-dihydroxy-5-(3-methyl-2-butenyl)benzoate showed significant trypanocidal activity, with IC50 values of 16.4, 15.6, and 18.5 µg mL-1, respectively. 2',6'-Dihydroxy-4'-methoxychalcone (DMC) purified from the dichloromethane extract of Piper aduncum inflorescences was active, in in vitro assays, against promastigotes and intracellular amastigotes of Leishmania amazonensis, with IC50 of 0.5 and 24 µg mL-1, respectively.107,108 In our laboratory, the screening for compounds with antileishmanial activity from different Piper species, P. permucromatum, P. tuberculatum, P. hispidum and P. renintens has proved worthwhile in bioassays against promastigote forms of Leishmania spp. (paper in preparation). Despite the effort of some research groups to study natural products obtained from the Amazonian forest, we could not find subsequent studies of clinical trials reported in the literature reviwed regarding the above compounds.
1.6 Peptides from Amazonian anuran fauna
The Amazon forest includes the highest number of the anuran species of the world.109 The anuran skin presents morphofunctional and behavioral protective adaptations against a number of adverse factors in the terrestrial environment.110 The venom gland produces noxious or toxic secretions, which are rich in substances with a variety of pharmacological effects.110 The complex chemical composition of the anuran skin secretions constitutes a source of biologically active compounds against bacteria, fungi and protozoa.111 Many of these molecules are known as antimicrobial peptides (AMPs). The emerging drug resistance of pathogenic microorganisms has stimulated interest in the development of antimicrobial peptides from anuran skin as therapeutic agents.111 Antimicrobial peptides compose the innate immunity system of anurans against microbial invasion.112-114 Crafted by evolution into an extremely diversified array of sequences and folds, AMPs do share a common amphiphilic 3-D arrangement.114 This feature is directly linked to a common mechanism of action that predominantly develops upon interaction of peptides with cell membranes of target cells.114 The mechanisms of action of AMPs in parasite membranes are complex and unknown, but they constitute a promising and attractive proposition as new antileishmanial and antimalarial therapeutics. The AMP mechanisms of interaction with the membrane are very complex. This complexity inhibits a fast adaptation of parasites, requiring a change in its membrane structure or composition, in contrast to drugs of intracellular action.115
Several anuran AMPs had leishmanicidal activity against different species of Leishmania (Table 1).117,118 These include the skin peptide YY (Skin-PYY) from Phyllomedusa bicolor (Amazon region),119 dermaseptin hypo01 (DShypo 01) from Phyllomedusa hypochondrialis (South America including Amazon region),120 dermaseptin S4 (DS IV) from Phyllomedusa sauvagii (Chacoan region) and its derivatives,121-123 dermasepin 01 (DS 01) from Phyllomedusa oreades (Cerrado region, Brazil),120 magainin 2 from Xenopus laevis (Central and south Africa),124 and temporins A and B from Rana temporaria (Europe).125 Skin polypetide YY from Amazon P. bicolor skin reduces the viability of promastigote forms of Leishmania major after one hour incubation at 25 µg mL-1.119 Dermaseptin hypo01 from Amazon P. hypochondrialis skin reduce to non-detectable levels the promastigote forms of Leishmania amazonensis at concentrations of near 64 µg mL-1 after 2 and 6 hour of incubation.120 These results clearly indicate an increased interest in AMPs with leishmanicidal activity. Moreover, the anuran AMP dermaseptin S4 and its derivatives were the only anuran AMPs tested in vitro against Plasmodium falciparum displaying a considerable effectiveness (IC50 of 7.7 µmol L-1 for dermaseptin S4 and 5.3 µmol L-1 for the derivate NC7-P at the ring stage, and 3.4 µmol L-1 and 6.2 µmol L-1, respectively, for the trophozoite stage).126 Dermaseptin S4 kills the intraerythrocytic malaria parasites through the lysis of the host cells, but its derivatives kill the parasite without lysing the erythrocyte.126,127 However, none of these peptides have been tested in vivo or against naturally acquired leishmaniasis or malaria.
1.7 The complexity of new chemical entity (NCE) development through Amazonian biodiversity
Designing a new drug to market is a time-consuming and expensive process for pharmaceutical companies, which need to identify potential targets, screening and development in order to maintain a competitive edge: technologies that require specialized expertise and infrastructure that are largely concentrated in pharmaceutical industries and are lacking in malaria and leishmania endemic countries. Successful drug discovery efforts include biochemical, biophysical, genetic, pharmacological and immunological humoral and cytological approaches, targeting such processes as signal transduction, cell cycle control, apoptosis, gene regulation, metastasis, and for malaria and leishmaniasis, the cell toxicity is highly relevant. The modern approaches for drug development combines a widespread use of combinatorial chemistry,128,129 high-throughput screening (HTS)130 associated with bioinformatics,131 crystallographic data132 and molecular-molecular docking interaction.133 The HTS assays could be accomplished from synthetic molecules already registered in data banks134-138 or natural products (extracts or purified molecules) from biodiversity.19,139 In spite of the traditional impact of naturally derived medicines and the incredible success stories of natural products (NPs) as drugs from the commencement of human therapeutic activity to modern research and drug development, most large pharmaceutical companies have scaled down or terminated their work in natural products research.140 Some authors, based on statistical data, argue that the rational search of NPs from biodiversity is more advantageous than searching synthetic molecular data bases138-142 since they often display both unique biological properties and a challenging structural complexity. For example, the broad capacity of secondary metabolism molecules found in the plants and animal venoms have provided defense from infectious attacks of microorganisms for thousands of years.143
Drug design by combinatorial chemistry and HTS created a large demand for small organic molecules that act on specific drug targets. These technologies focus on the generation of a huge number of molecules integrated with biological screening from a very large number of samples.137 An increasing pressure to reduce drug development time and cost by the pharmaceutical industries has stimulated the search for relevant new molecules for commercially viable diseases such as cancer, cardiovascular, neural diseases and hospital infections but not for neglected diseases, which are not profitable. Even so, the pathway to be covered to validate a new drug from a new chemical entity (NCE) is exhaustive. In order to make it, the NCE must pass through a series of hurdles. The initial set of hurdles to overcome is passing from the different drug discovery stages to the preclinical phase (see Table 2).144 Combinatorial chemistry and HTS are the technologies necessary to overcome the discovery phase obstacles listed in Table 2. Unfortunately, these technologies are well implemented mainly in the pharmaceutical industries of developed countries, or are restricted to some Universities and Research Institutes.
The huge biodiversity within the Brazilian territory puts the country in a strategic position to rationally and sustainably explore NCEs for malaria, leishmaniasis and many other neglected or chronic diseases that possess high pharmaceutical commercial speculation. In the future, perspectives for drug design for malaria and leishmaniasis should focus on searching for specific parasite metabolic route inhibition through plant extracts and animal venoms or secretions screening by HTS and combinatorial chemistry. The basis for sustained exploration of biodiversity relies on clonal propagations, seed banks for preservation of genetic diversity145,146 or sustained animal contentions in captivity, i.e., if a plant/animal extract strongly binds to an enzymatic target, studies on the propagation of the species to supply biomass for extraction, fractionation and purification of the active metabolite(s) must initiate as soon as possible. However, the Amazonian biodiversity is poorly explored for NCE development. One of the reasons for this poor exploration is the lack of enough research institutions and qualified scientists in the Amazon region. This structural problem associated with the biodiversity access legal bureaucracy, mainly in national reserves and indigenous nation's areas, creates strong hurdles to scientific exploration and development of the NP potential of the Amazon forest.
2. Final Considerations
Amazonian biomolecular diversity has a huge potential as a source of molecules with biological activity against Leishmania sp and Plasmodium sp. But it still remains an underexplored resource. This fact is evidenced by the large-scale identification of compounds with antileishmanic and antimalaric activity from animal and plant species in other biomes of the world with minor biodiversity while Amazonian correlated species remain without research. But why does this region remain as an underexplored biomolecular source? The answer to why the scientific approach is incompatible with the volume of species in the Amazon region relates to geographical factors, and structural and legal problems. The Amazon region encompasses seven million square kilometers (1.7 billion acres), of which five and a half million square kilometers (1.4 billion acres) are covered by the rainforest. This region includes territory belonging to nine nations and many areas are of difficult access and residence. Among the structural problems is the number of researchers in the Amazon: according to the Coordenação de Aperfeiçoamento de Pessoal de Nível Superior (CAPES/MEC), up to 2005 only one thousand PhDs were working in the Brazilian Amazon area,146 very few in proportion to the size of the area, which represents 60% of Brazilian territory. The number of properly equipped laboratories is even smaller, probably not more than 20 to 50. The third problem to the strong barrier against the development of research in the Amazon is the Brazilian legal framework. The legal bureaucracy discourages and chases away scientific initiatives in the region. This problem is so serious that it prevents the implementation of scientific projects funded from the government budget and implemented by officials of the government itself. To reverse this situation concerning the molecular diversity description of the Amazonian rainforest, as a way of subsidizing the development of drugs to be applied to leishmaniasis and malaria, it is necessary to associate conventional phytochemistry methods, HTS and combinatorial chemistry from both research institutes and industry and they must be supported by rational governmental policies including financial and legal efforts to minimize the structural and bureaucratic barriers.
The creation of the Biodiversity and Biotechnology Web of the Legal Amazonia (Rede Bionorte) by the Brazilian Minister of Science e Technology is a very good example of public policy for Amazonian NCE exploration. This web has the objective of coordinating and generating financial support for research projects to be developed in Amazonia only by existing regional institutions and research groups. The incentive politics to finance projects can be the first step to stimulate the scientific development of this region through the implantation of new institutions of research and the reinforcement of those already existing. The Bionorte web is an important way of Amazonian biodiversity preservation and its model should be adjusted for others strategic scientific and political areas for Amazonian development.
Acknowledgments
The authors are grateful to the Ministry of Science and Technology (MCT), CNPq and FINEP and to the Secretary of Development of the Rondonia State (PRONEX/CNPq) for financial support.
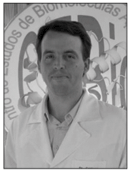
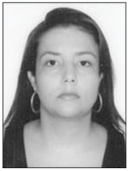
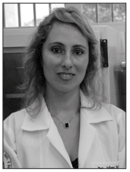
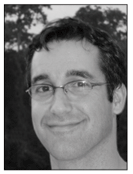
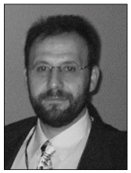
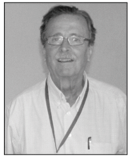
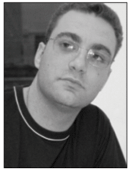
Received: February 2, 2009
Web Release Date: June 26, 2009
FAPESP helped in meeting the publication costs of this article.
- 1. Olson, D. M.; Dinerstein, E.; Conserv. Biol. 1998, 12, 502.
- 2. Myers, N.; Mittermeier, R. A.; Mittermeier, C. G.; Fonseca, G. A. B.; Kent, J.; Nature 2000, 403, 853.
- 3. Olson, D. M.; Dinerstein, E.; Wikramanayake, E. D.; Burgess, N. D.; Powell, G. V. N.; Underwood, E. C.; D'Amico, J. A.; Itoua, I.; Strand, H. E.; Morrison, J. C.; Loucks, C. J.; Allnutt, T. F.; Ricketts, T. H.; Kura, Y.; Lamoreux, J. F.; Wettengel, W. W.; Hedao, P.; Kassem, K. R.; BioScience 2001, 51, 933.
- 4. Turner, I. M.; The Ecology of Trees in the Tropical Rain Forest; Cambridge University Press: Cambridge, UK, 2001.
- 5. Mittermeier, R. A. In Biodiversity; Wilson, E. O., ed.; National Academy Press: Washington, United State of America, 1988.
-
6http://www.worldwildlife.org/wildplaces/amazon/index.cfm, accessed in May 2008.
» link - 7. McNeely, J. A.; Miller, K. R.; Reid, W. V.; Mittermeier, R. A.; Werner, T. B.; Conserving the World's Biological Diversity; IUCN: Gland, Switzerland, 1990.
- 8. Erwin, T. L.; Biodiversity; Wilson, E. O., ed.; National Academy Press: Washington, 1988.
- 9. Myers, N.; Mittermeier, R. A.; Mittermeier, C. G.; Fonseca, G. A. B.; Kent, J.; Nature 2000, 403, 853.
- 10. Silva, J. M. C.; Rylands, A. B.; Fonseca, G. A. B.; Conserv. Biol. 2005, 19, 689.
- 11. Hopkins, M. J. G.; J. Biogeogr. 2007, 34, 1400.
- 12. Lorenzi, H.; Matos, F. J. A.; Plantas Medicinais no Brasil: Nativas e Exóticas Instituto Plantarum de Estudos da Flora: São Paulo, Brasil, 2000.
- 13. Harvey, A. L.; Drug Discov. Today 2008, 13, 894.
- 14. Sneader, W.; Drug Prototypes and Their Exploitation, Wiley: Chichester, United Kingdom, 1996.
- 15. Rishton, G. M.; Am. J. Cardiol. 2008, 101, 43D.
- 16. Stockwell, B. R.; Nature 2004, 432, 846.
- 17. Newman, D. J.; Cragg, G. M.; Snader, K. M.; J. Nat. Prod. 2003, 66, 1022.
- 18. Butler, M. S.; Nat. Prod. Rep. 2008, 25, 475.
- 19. Newman, D. J.; Cragg, G. M.; J. Nat. Prod 2007, 70, 461.
- 20. Bourdy, G.; Willcox, M. L.; Ginsburg, H.; Rasoanaivo, P.; Graz, B.; Deharo. E.; Int. J. Parasitol. 2008, 38, 33.
- 21. Ockenhouse, C. F.; Magill, A.; Smith, D.; Milhous, W.; Mil. Med. 2005, 170, 12.
- 22. Lee, M. R.; J. R. Coll. Physicians Edinb. 2002, 32, 189.
- 23. Kitchner, I. S.; Infec. Dis. 2003, 4, 34.
- 24. Santos, A. O.; Ueda-Nakamura, T.; Dias Filho, B. P.; Veiga Junior, V. F.; Pinto, A. C.; Nakamura, C. V.; J . Ethnopharmacol. 2008, 120, 204.
- 25. Alves, R. R. N.; Rosa, I. M. L.; J. Ethnobiol. Ethnomed. 2007, 3, 14.
- 26. Tempone, A. G.; Sartorelli, P.; Teixeira, D.; Prado, F. O.; Calixto, I. A. R. L; Lorenzi, H.; Melhem, M. S. C.; Mem. Inst. Oswaldo Cruz 2008, 103, 443.
- 27. Bourdy, G.; Willcox, M. L.; Ginsburg, H.; Rasoanaivo, P.; Graz, B.; Deharo, E.; Int. J. Parasitol. 2008, 38, 33.
- 28. Abu-Raddad, L. J.; Patnaik, P.; Kublin, J. G.; Science 2006, 314, 1603.
- 29. Guinovart, C.; Navia, M. M.; Tanner, M.; Alonso, P. L.; Curr. Mol. Med. 2006, 6, 137.
- 30. Herwaldt, B. L.; Lancet 1999, 354, 1191.
- 31. Murray, H. W.; Berman, J. D.; Davies, C. R.; Saravia, N. G.; Lancet 2005, 366, 1561.
- 32. Berman, J. D.; Clin. Infect. Dis. 1997, 24, 684.
- 33. Pearson, R. D.; Sousa, A. Q.; Clin. Infect. Dis. 1996, 22, 1.
- 34. Desjeux, P.; Clin. Dermatol. 1996, 14, 417.
- 35. Foster, S. D. F. Malaria: waiting for the vaccine; Targett, G. A. T., ed.; John Wiley: Chichester, UK,1991.
- 36. Wallace, D. J.; Semin. Arthritis Rheum. 1989, 18, 282.
- 37. Silva, T. H. A.; Oliveira, M. T.; dos Santos, H. F.; de Oliveira, A. B.; de Almeida, W. B.; Quim. Nova 2005, 28, 244.
- 38. Robert, A.; Benoii-Vical, F.; Dechy-Cabaret, O.; Meunier, B.; Pure Appl. Chem. 2001, 73, 1173.
- 39. WHO. In The Use of Antimalarial Drugs; Poole, S. ed.; WHO & Graficim: Geneva, Switzerland, 2001.
- 40. Boss, C.; Richard-Bildstein, S.; Weller, T.; Fischli, W.; Meyer, S.; Binkert, C.; Curr. Med. Chem. 2003, 10, 883.
- 41. Nzila, A.; J. Antimicrob. Chemother. 2006, 57, 1043.
- 42. Phillips, R. S.; Clin. Microbiol. Rev. 2001, 14, 208.
- 43. Foley, M.; Tilley, L.; Pharmacol. Ther. 1998, 79, 55.
- 44. Boudreau, E. F.; Webster, H. K.; Pavanand, K.; Thosingha, L.; Lancet 1982, 2, 1335.
- 45. Bygbjerg, I. C.; Schapira, A.; Flachs, H.; Gomme, G.; Jepsen, S.; Lancet 1983, 1, 774.
- 46. Nateghpour, M.; Ward, S. A.; Howells, R. E.; Antimicrob. Agents Chemother. 1993, 37, 2337.
- 47. Croft, S. L.; Coombs, G. H.; Trends Parasitol. 2003, 19, 502.
- 48. Sundar, S.; More, D. K.; Singh, M. K.; Singh, V. P.; Sharma, S.; Makharia, A.; Kumar, P. C.; Murray, H. W.; Clin. Infect. Dis. 2000, 31, 1104.
- 49. Sundar, S.; Trop. Med. Int. Health 2001, 6, 849.
- 50. Davis, A. J.; Kedzierski, L.; Curr. Opin. Investig. Drugs 2005, 6, 163.
- 51. Murray, H. W.; Expert. Rev. Anti-Infect. Ther. 2004, 2, 279.
- 52. Berman, J. D.; Badaró, R.; Thakur, C. P.; Wasunna, K. M.; Behbehani, K.; Davidson, R.; Kuzoe, F.; Pang, L.; Weerasuriya, K.; Bryceson, A. D.; Bull. World Health Organ. 1998, 76, 25.
- 53. Cragg, G. M.; Newman, D. J.; Snader, K. M.; J. Nat. Prod. 1997, 60, 52.
- 54. Newman D. J.; Cragg, G. M.; Snader, K. M.; J. Nat. Prod. 2003, 66, 1022.
- 55. Guo, Z.; Vangapandu. S.; Sindelar R. W.; Walker, L. A.; Sindelar, R. D.; Curr. Med. Chem. 2005, 12, 173.
- 56. Barbosa-Filho, J. M.; Piuvezam, M. R.; Moura, M. D.; Silva, M. S.; Lima, K. V. B.; Cunha, E. V. L.; Fechine, I. M.; Takemura, O. S.; Rev. Bras. Farmacogn. 2006, 16, 109.
- 57. Harborne, J. B.; The Chemical Basis of Plant Defense, CRC Press: New York, United State of America, 1991.
- 58. Osorio, E. J.; Robledo, S. M.; Bastida, J.; Alkaloids. Chem. Biol. 2008, 66, 113.
- 59. Mishra, B. B.; Kale, R. R.; Singh, R. K., Tiwari, V. K.; Fitoterapia, 2009, 80, 149.
- 60. Holetz, F. B.; Pessini, G. L.; Sanches, N. R.; Cortez, D. A. G.; Nakamura, C. V.; Dias Filho, B. P.; Mem. Inst. Oswaldo Cruz 2002, 97, 1027.
- 61. Salatino, A.; Salatino, M. L. F.; Negri, G.; J. Braz. Chem. Soc. 2007, 18, 11.
- 62. Andrade-Neto, V. F.; Pohlit, A. M.; Pinto, A. C. S.; Silva, E. C. C.; Nogueira, K. L.; Melo, M. R. S.; Henrique, M. C.; Amorim, R. C. N.; Silva, L. F. R.; Costa, M. R. F.; Nunomura, R. C. S.; Nunomura, S. M.; Alecrim, W. D.; Alecrim, M. G. C.; Chaves, F. C. M.; Vieira, P. P. R.; Mem. Inst. Oswaldo Cruz 2007, 102, 359.
- 63. Andrade-Neto, V. F.; Brandão, M. G. L.; Oliveira, F. Q.; Casali, V. W. D.; Njaine, B.; Zalis, M. G.; Oliveira, L. A.; Krettli, A. U.; Phytother. Res. 2004, 18, 634.
- 64. Andrade-Neto, V. F.; Brandão, M. G.; Stehmann, J. R.; Oliveira, L.A.; Krettli A. U.; J. Ethnopharmacol. 2003, 87, 253.
- 65. Garavito, G.; Rincón, J.; Arteaga. L.; Hata, Y.; Bourdy, G.; Gimenez, A.; Pinzón, R.; Deharo, E.; J Ethnopharmacol 2006, 107, 460.
- 66. Yenesew, A.; Derese. S.; Irungu. B.; Midiwo, J. O.; Waters, N. C.; Liyala, P.; Akala, H.; Heydenreich. M.; Peter, M. G.; Planta Med. 2003, 69, 658.
- 67. Flores, N.; Jiménez, I. A.; Giménez, A.; Ruiz, G.; Gutiérrez, D.; Bourdy, G.; Bazzocchi, I. L.; J. Nat. Prod. 2008, 71, 1538.
- 68. Amorim, C. Z.; Flores, C. A.; Gomes, B. E.; Marques, A. D.; Cordeiro, R. S. B.; J. Ethnopharmacol. 1988, 24, 101.
- 69. Amorim, C. Z.; Gomes, B. E.; Flores, C. A.; Cordeiro, R. S. B.; Braz. J. Med. Biol. Res. 1986, 19, 569.
- 70. Almeida, M. M. B.; Arriaga, A. M. C.; Santos, A. K. L.; Lemos, T. L. G.; Braz-Filho, R.; Vieira, I. J. C.; Quim. Nova 2007, 30, 935.
- 71. Bertania. S.; Houëlb, E.; Stienb, D.; Chevolotc, L.; Julliand, V.; Garavitoe, G.; Bourdyf G.; Deharo, E.; J. Ethnopharmacol. 2006, 108, 155.
- 72. Muhammad, I.; Bedir, E.; Khan, S. I.; Tekwani, B. L.; Khan, I. A.; Takamatsu, S.; Pelletier, J.; Walker, L. A.; J. Nat. Prod 2004, 67, 772.
- 73. Kitagawa, I.; Mahmud, T.; Yokota, K.; Nakagawa, S.; Mayumi, T.; Kobayashi, M.; Shibuya, H.; Chem. Pharm. Bull. 1996, 44, 2009.
- 74. Andrade-Neto, V. F.; Goulart, M. O. F.; Silva Filho, J. F.; da Silva, M. T.; Pinto, M. C. F. R.; Pinto, A. V.; Zalis, M. G.; Carvalho, L. H.; Krettli, A. U.; Bioorg. Med. Chem. Lett. 2004, 14, 1145.
- 75. Ferreira, M. E.; Nakayama, H.; de Arias, A. R.; Schinini, A.; de Bilbao, N. V.; Serna, E.; Lagoutte, D.; Soriano-Agaton, F.; Poupon, E.; Hocquemiller, R.; Fournet, A.; J. Ethnopharmacol. 2006, 109, 258.
- 76. Costa, E. V.; Pinheiro, M. L. B.; Xavier, C. M.; Silva, J. R. A.; Amaral, A. C. F.; Souza, A. D. L.; Barison, A.; Campos, F. R.; Ferreira, A. G.; Machado, G. M. C.; Leon, L. P. L.; J. Nat. Prod. 2006, 69, 292.
- 77. Brenzan, M. A.; Nakamura, C. V.; Dias Filho, B P.; Ueda-Nakamura, T.; Young, M. C.; Cortez, D.A. G; Parasitol. Res. 2007, 101,715.
- 78. Sartorelli, P.; Andrade, S. P.; Melhem, M. S.; Prado, F. O.; Tempone, A. G.; Phytother. Res. 2007, 21, 644.
- 79. Santos, A. O.; Ueda-Nakamura, T.; Dias-Filho, B. P.; Veiga Jr, V. F.; Pinto, A. C.; Nakamura, C. V.; J. Ethnopharmacol 2008, 120, 204.
- 80. Rosa, M. S. S.; Mendonça-Filho, R. R.; Bizzo, H. R.; Rodrigues, I. A.; Soares, R. M.; Padrón, T. S.; Alviano, C. S.; Lopes, A. H. C. S.; Antimicrob. Agents Chemother. 2003, 47, 1895.
- 81. Correa, J. E.; Ríos, C. H.; del Rosario Castillo, A.; Romero, L. I.; Ortega-Barría, E.; Coley, P. D.; Kursar, T. A.; Heller, M. V.; Gerwick, W. H.; Rios, L. C.; Planta Med. 2006, 72, 270.
- 82. Passero, L.; Castro, A.; Tomokane, T.; Kato, M.; Paulinetti, T.; Corbett, C.; Laurenti, M.; Parasitol. Res. 2007, 101, 677.
- 83. Nakamura, C. V.; Santos, A. O.; Vendrametto, M. C.; Luize, P. S.; Filho, B. P. D.; Cortez, D. A. G.; Ueda-Nakamura, T.; Rev. Bras. Farmacogn. 2006, 16, 61.
- 84. Moreira, R. C. R.; Costa, G. C.; Lopes, T. C.; Bezerra, J. L.; Guerra, R. N. M.; Rebêlo, J. M. M.; Ribeiro, M. N. S.; Nascimento, F. R. F.; Costa, J. M. L.; Rev. Bras. Farmacogn. 2007, 17, 59.
- 85. Torres-Santos, E. C.; Lopes, D.; Rodrigues Oliveira, R.; Carauta, J. P. P; Bandeira Falcao C. A.; Kaplan, M. A. C.; Rossi-Bergmann, B.; Phytomedicine, 2004, 114.
- 86. Phillipson J. D.; Wright, C. W.; Trans. R. Soc. Trop. Med. Hyg. 1991, 85, 18.
- 87. Chan-Bacab, M. J.; Pena-Rodriguez, L. M.; Nat. Prod. Rep. 2001, 18, 674.
- 88. Queiroz, E. F.; Roblot, F.; Cavé, A.; Paulo, M. Q.; Fournet, A.; J. Nat. Prod 1996, 59, 438.
- 89. Mahiou, V.; Roblot, F.; Hocquemiller, R.; Cavé, A.; Rojas de Arias, A.; Yaluff, G.; Fournet, A.; Angelo, A.; J. Nat. Prod. 1994, 57, 890.
- 90. Montenegro, H.; Gutiérrez, M.; Romero, LI.; Ortega-Barría, E.; Capson, T. L.; Rios, L. C.; Planta. Med. 2003, 69, 677.
- 91. Maciel, M. A. M.; Pinto, A. C.; Veiga Jr, V. F.; Grynberg, N. F.; Echevarria, A.; Quim. Nova 2002, 25, 429.
- 92. Veiga Jr, V. F.; Pinto, A. C.; Quim. Nova 2002, 25, 273.
- 93. Basile, A. C.; Sertié, J. A.; Freitas, P. C.; Zanini, A. C.; J. Ethnopharmacol 1988, 22, 101.
- 94. Parmar, V. S.; Jain, S. C.; Bisht, K. S.; Jain, R.; Taneja, P.; Jha, A.; Tyagi, O. M.; Prasad, A. K.; Wengel, J.; Olsen, C. E.; Boll, P.; Phytochemistry 1997, 46, 597.
- 95. Facundo, V. A.; Morais, S. M.; Biochem. Syst. Ecol 2003, 31, 111.
- 96. Facundo, V. A.; Braz-Filho, R.; Biochem. Syst. Ecol 2004, 33, 1215.
- 97. Facundo, V. A.; Sá, A. L.; Silva, S. A. F.; Morais, S. M.; Matos, C. R. R.; Braz-Filho, R.; J. Braz. Chem. Soc. 2004, 15, 140.
- 98. Facundo, V. A.; Silveira, A. S. P.; Morais, S. M.; Biochem. Syst. Ecol 2005, 33, 753.
- 99. Facundo, V. A.; Morais, S. M.; J. Essent. Oil Res 2005, 17, 304.
- 100. Parmar, V. S.; Jain, S. C.; Gupta, S.; Talwar, S.; Rajwanshi, V. K.; Kumar, R.; Azin, A.; Malhotra, S.; Kumar, N.; Jain, R.; Nawal, K. S.; Tyagi, O. M.; Lawrie, S. J.; Errington, W.; Howarth, O. W.; Olsen, C.E.; Singh, S. K.; Wengel, H.; Phytochemistry 1998, 49, 1069.
- 101. Rukachaisirikul, T.; Siriwattanakit, K. S.; Wongvein, C.; Ruttanaweang, P.; Wongwattanavuch, P.; Suksamrarn, A.; J. Ethnopharmacol. 2004, 93, 173.
- 102. Lin, L. C.; Shen, C. C.; Shen, Y. C.; Tsai, T. H.; J. Nat. Prod. 2006, 69, 842.
- 103. Pessine, G. L.; Dias-Filho, B. P.; Nakamura, C. V.; Cortez, D. A. G.; Mem. Inst. Oswaldo Cruz 2003, 98, 1115.
- 104. Pessine, G. L.; Dias-Filho, B. P.; Nakamura, C. V.; Cortez, D. A. G.; J. Braz. Chem. Soc. 2005, 16, 1130.
- 105. Chauret, D. C.; Bernard, C. B.; Arnason, J. T.; Durst, T.; J. Nat. Prod. 1996, 59, 152.
- 106. Torres-Santos, E. C.; Moreira, D. L.; Kaplan, M. A. C.; Meireles, M. N.; Rossi-Bergmann, B.; Antimicrob. Agents Chemother. 1999, 43, 1234.
- 107. Torres-Santos, E. C.; Rodrigues, J. M.; Moreira, D. L.; Kaplan, M. A. C.; Rossi-Bergmann, B.; Antimicrob. Agents Chemother. 1999, 43, 1776.
- 108. Silvano, D. L.; Segalla, M. V.; Conserv. Biol. 2005, 19, 653.
- 109. Toledo, R. C.; Jared, C.; Comp. Biochem. Physiol.: Part A 1995, 11, 1.
- 110. Barra, D.; Simmaco, M.; Trends Biotechnol. 1995, 13, 205.
- 111. Rinaldi, A.C.; Curr. Opin. Chem. Biol 2002, 6, 799.
- 112. Zasloff, M.; Nature 2002, 415, 389.
- 113. Radek, K.; Gallo, G.; Semin. Immunopathol. 2007, 29, 27.
- 114. Giuliani, A.; Pirri, G.; Bozzi, A.; Di Giulio, A.; Aschi, M.; Rinaldi, A. C.; Cell. Mol. Life Sci. 2008, 65, 2450.
- 115. Phillips, R. S.; Clin. Microbiol. Rev. 2001, 14, 208.
- 116. Davis, A. J.; Kedzierski, L.; Curr. Opin. Investig. Drugs 2005, 6, 163.
- 117. Alberola, J.; Rodríguez, A.; Francino, O.; Roura, X.; Rivas, L.; Andreu, D.; Antimicrob. Agents Chemother. 2004, 48, 641.
- 118. Vouldoukis, I.; Shai, Y.; Nicolas, P.; Mor, A.; FEBS Lett. 1996, 380, 237.
- 119. Brand, G. D.; Leite, J. R. S. A.; De Sa Mandel, S. M.; Mesquita, D. A.; Silva, L. P.; Prates, M. V.; Barbosa, E. A.; Vinecky, F.; Martins, G. R.; Galasso, J. H.; Kuckelhaus, S. A.; Sampaio, R. N.; Furtado, J. R. Jr.; Andrade, A. C.; Bloch, C. Jr.; Biochem. Biophys. Res. Commun. 2006, 347, 739.
- 120. Feder, R.; Dagan, A.; Mor, A.; J. Biol. Chem. 2000, 275, 4230.
- 121. Kustanovich, I.; Shalev, D. E.; Mikhlin, M.; Gaidukov, L.; Mor, A.; J. Biol. Chem. 2002, 277, 16941.
- 122. Gaidukov, L.; Fish. A.; Mor, A.; Biochemistry 2003, 42, 12866.
- 123. Guerrero, E.; Saugar, J. M.; Matsuzaki, K.; Rivas, L.; Antimicrob. Agents Chemother. 2004, 48, 2980.
- 124. Mangoni, M. L.; Saugar, J. M.; Dellisanti, M.; Barra, D.; Simmaco, M.; Rivas, L.; J. Biol. Chem. 2005, 280, 984.
- 125. Dagan, A.; Efron, L.; Gaidukov, L.; Mor, A.; Ginsburg, H.; Antimicrob. Agents Chemother. 2002, 46, 1059.
- 126. Efron, L.; Dagan, A.; Gaidukov, L.; Ginsburg, H.; Mor, A.; J. Biol. Chem. 2002, 277, 24067.
- 127. Maclean, D.; Baldwin, J. J.; Ivanov, V. T.; Kato, Y.; Shaw, A.; Schenider, P.; Gordon, E. M.; J. Comb. Chem. 2000, 2, 562.
- 128. Amaral, P.A.; Neves, G.; Farias, F.; Eifler-Lima, V. L.; Rev. Bras. Cienc. Farm. 2003, 39, 351.
- 129. Liu, B.; Li, S.; Hu, J.; Am. J. Pharmacogenom. 2004, 4, 263.
- 130. Poulain, R.; Horvath, D.; Bonnet, B.; Eckhoff, C.; Chapelain, B.; Bodinier, M. C.; Deprez, B.; J. Med. Chem. 2001, 44, 3391.
- 131. Chapman, T.; Nature 2004, 430, 109.
- 132. Kitchen, D. B.; Decornez, H.; Furr, J. R.; Bajorath, J.; Nat. Rev. Drug Discovery 2004, 3, 935.
- 133. Maclean, D.; Baldwin, J. J.; Ivanov, V. T.; Kato, Y.; Shaw, A.; Schenider, P.; Gordon, E. M.; J. Comb. Chem. 2000, 2, 562.
- 134. Poulain, R.; Horvath, D.; Bonnet, B.; Eckhoff, C.; Chapelain, B.; Bodinier, M. C.; Deprez, B.; J. Med. Chem. 2001, 44, 3391.
- 135. Horton, D. A.; Bourne, G. T.; Smythe, M. L.; Chem. Rev. 2003, 103, 893.
- 136. Deprez-Poulain, R.; Deprez, B.; Front. Med. Chem 2006, 3, 653.
- 137. Beghyn, T.; Deprez-Poulain, R.; Willand, N.; Folleas, B.; Deprez, B.; Chem. Biol. Drug Des. 2008, 72, 3.
- 138. Ortholand, J.; Ganesan, A.; Curr. Opin. Chem. Biol. 2004, 8, 271.
- 139. Rollinger, J. M.; Langer, T.; Stuppner, H.; Curr. Med. Chem 2006, 13, 1491.
- 140. Tulp, M.; Bohlin, L.; Trends Pharmacol. Sci. 2002, 23, 225.
- 141. Koehn, F. E.; Carter, G. T.; Nat. Rev. Drug Discovery 2005, 4, 206.
- 142. Wallace, R. J.; Proc. Nutr. Soc. 2004, 63, 621.
- 143. Nwaka, S.; TDR Scientific Working Group on Malaria, Geneva, Switzerland, 2003.
- 144. Clardy, J.; Walsh, C.; Nature 2004, 432, 829.
- 145. Basso, L. A.; Pereira da Silva, L. H.; Fett-Neto, A. G.; Azevedo Junior, W. A.; Beghyn T.; Deprez-Poulain, R.; Willand, N.; Folleas, B., Deprez, B.; Chem. Biol. Drug Des 2008, 72, 3.
-
146http://www.capes.gov.br/servicos/sala-de-imprensa/36-noticias/1249, accessed in May 2009.
» link
Publication Dates
-
Publication in this collection
04 Aug 2009 -
Date of issue
2009
History
-
Accepted
26 June 2009 -
Received
02 Feb 2009