Abstract
Ion exchange isotherms are very important tools to achieve a better comprehension of cation removal by means of zeolite treatment. In this work, three isotherms were obtained (at 298K, at 313K and at 333K) from natural pretreated Na+ clinoptilolite. The ion exchange was carried out with Cr+3 ions. The isotherms’ shape is similar to the classical type "b" isotherm, according to the arrangement proposed by Breck (1984). Mathematical fitting was applied to the experimental points (Table Curve software) to obtain a representative curve thereof. From such fittings, points were simulated and then used to construct the Kielland plots, whose shape was associated with an ion exchange mechanism. Straight lines were obtained as an indication that, although the zeolite used is of natural occurrence and presents impurities such as mordenite and clays, only one site is involved in the ion exchange process
Clinoptilolite; ion exchange mechanism; kielland plot
ION EXCHANGE MECHANISM OF Cr+3 ON NATURALLY OCCURRING CLINOPTILOLITE
M.A.S.D. de Barros 1 , N.R.C.F. Machado 1 , F.V. Alves 1 and E.F. Sousa-Aguiar 2,3
1Universidade Estadual de Maringá, Depto de Engenharia Química, Av. Colombo 5790, Bloco D-90,
CEP 87020-900, Maringá-PR. FAX: 55-44-2633440, nadia@cybertelecom.com.br
2CENPES/PETROBRÁS - DICAT
3Escola de Química - UFRJ - DPO
(Received: March 5, 1997; Accepted: August 5, 1997)
Abstract: Ion exchange isotherms are very important tools to achieve a better comprehension of cation removal by means of zeolite treatment. In this work, three isotherms were obtained (at 298K, at 313K and at 333K) from natural pretreated Na+ clinoptilolite. The ion exchange was carried out with Cr+3 ions. The isotherms shape is similar to the classical type "b" isotherm, according to the arrangement proposed by Breck (1984). Mathematical fitting was applied to the experimental points (Table Curve software) to obtain a representative curve thereof. From such fittings, points were simulated and then used to construct the Kielland plots, whose shape was associated with an ion exchange mechanism. Straight lines were obtained as an indication that, although the zeolite used is of natural occurrence and presents impurities such as mordenite and clays, only one site is involved in the ion exchange process.
Keywords: Clinoptilolite, ion exchange mechanism, kielland plot.
INTRODUCTION
Zeolites are crystalline aluminosilicates from natural or synthetics sourcir. The physical structure is porous with interconnected cavities in which metal cations and water molecules are contained. The zeolites have reversible hydration properties in addition to their cation exchange properties. The fundamental building block of the zeolites is a tetrahedron of four oxygen atoms surrounding a relatively small silicon or aluminum atom. The structure consists of SiO4 and AlO4 tetrahedra arranged so that each oxygen atom is shared between two tetrahedra. Because aluminum has one less positive charge than silicon, the framework has a net negative charge of one at the site of each aluminum atom and is balanced by the exchangeable cation. Substitution of Al+3 for other cations is possible mainly in natural zeolites. Samples containing Ga, Ge and Fe instead of Al are also likely to be found.
The ion exchange property has been used to study the exchange behaviour between a zeolitic system containing Na, Ca and K and Cr ions initially in solution. For this purpose, a natural Cuban zeolite has been used. This mineral is formed by two kinds of zeolite: clinoptilolite, and mordenite.
Clinoptilolite is the most abundant natural zeolite (Ackley, 1992). This kind of zeolite was considered a new mineral by Schaller in 1923. Until then, clinoptilolite had been considered to be ptolite (mordenite). In 1934, Hey and Bannister showed that clinoptilolite could be considered to be a rich silicon heulandite. This mineral has a high thermal resistance. Heating up to 750ºC does not modify its original structure. This feature, according to Mumpton, is due to the high silicon concentration. Mason and Sand state that the thermal resistance of clinoptilolite is due to its main exchangeable cations (Na, K) which are monovalents (Alietti, 1972). Clinoptilolite belongs to the structural group 7 and has a monoclinic symmetry and a Si/Al ratio between 4.25 and 5.25. Czárán et al. (1988) declare that, despite the fact that the Si/Al ratio is close to 5 and the cationic exchange capacity (Al equivalents that belong to zeolite net) is 2.3 meq/100g, the experimentally obtained results can vary a lot. This feature is due to its natural occurrence. The authors also obtained a Si/Al ratio higher than 5, which indicates the presence of non-framework elements. It has been ascertained too, that the the sum of the existing cations in the zeolite is smaller than that of the Al equivalents. This indicates that, besides the non-framework alumina, non-exchangeable cations occurring as impurities are present.
Clinoplilolite's structure is presented by a series of interconnected channels. This bidimensional system is made of channels A (10-member ring) and B (8-member ring) that are parallel to one an other and to the c axis of the unit cell. The C channel (8-member ring) lies along the a axis intersecting both the A and B channels.
Mordenite is the richest silicon zeolite (Breck, 1984). Each tetrahedron belongs to one or more 5-member rings. This zeolite has a high thermal stability, probably a consequence of the great number of such rings, which are energetically favoured concerning stability. This zeolite belongs to the structural group 6, has an orthorombic symmetry and a Si/Al ratio between 4.17 and 5.0.
The ion exchange reaction may be written as
,
where cations A and B have ZA and ZB charges, respectively. The letters "c" and "s", are related to the crystalline phase (zeolite) and to the solution. This reaction expresses the ion exchange phenomenon that occurs in the mineral and depends on several factors. The ion exchange capacity is directly related to the quantity of aluminum present in the zeolite framework. In such a case, a low Si/Al ratio, or rather, a high aluminum concentration, favours this process. However, other parameters, such as the nature of the cationic species in solution and the anionic species associated to them, the solvent and the temperature must be observed for a good efficiency of exchange. Cations with high charges and small radii are preferred for the zeolite. However, cations with large radii, even those with high charges, may not be exchanged due to steric factors, since the zeolitic channels display molecular dimensions. The mordenite pore opening is (2.9 x 5.7-6.7 x 7.0Å) and that for the clinoptilolite is (4.0 x 5.5-4.4 x 7.2Å). The temperature also favours the exchange (Breck, 1984). The Cr+3 ion, in spite of having a high charge and a small size (0.64Å), has a strong tendency to undergo hydration (hydrated radii = 4.61Å). In this way, zeolite preference for this cation may be reduced due to a steric problem.
Figure 1: Clinoptilolite structure.
Figure 2: Mordenite structure.
XAS
AZ
Figure 3: Ion exchange isotherms described by Breck (1984).
Many authors (Barros et al., 1995) state that the natural zeolite, containing several exchangeable cations, needs a pretreatment, which tends to replace them by only one kind of cation. This procedure improves the zeolite exchange capacity, which means that more aluminum sites are capable of undergoing ion exchange.
The exchange behaviour can be shown through isotherms. These isotherms may be obtained graphically and present concentrations of a given ion, both in the zeolitic phase and in solution, in an equilibrium system. Therefore, the isotherms show whether or not the zeolite is favourable to the exchange. The isotherm may be plotted using the equivalent fraction of the cation A (originally in the liquid phase) in the solution (XAS) versus the equivalent fraction of the cation A in the zeolite (XAZ).
According to Breck (1984), five types of isotherms (see Figure 3) may be obtained. Type "a," with a selectivity for the entering cation over the entire range of the zeolite; type "b," in which the entering cation shows a selectivity reversal with an increasing equivalent fraction in the zeolite; type "c," with a selectivity for the exiting cation over the entire range of the zeolite composition; type "d," in which exchange is not completed although the entering cation is initially preferred, and type "e," in which hysteresis effects may result from the formation of two zeolite phases.
There is another arrangement presented by Townsend (1991). The isotherms are classified in four types. The isotherms of the first type are presented in Figure 4a and represent relatively simple systems. Case 1 shows that the zeolite does not prefer the ion that enters. Case 2 is obtained when the zeolite is not selective. Case 3 occurs when it is selective. Isotherms of the second type (Figure 4b) show a sigmoid shape and indicate a change in the selectivity as a function of XAZ. Isotherms of the third type (Figure 4c) present a plateau in the hysteresis region. This kind of behaviour is due to the formation of a new crystalline phase that occurred during the exchanging process, as in the isotherms type "e." Finally, isotherms of the fourth type (Figure 4d) are frequently observed for the zeolites. One can also observe an exchange limit which is inferior to the theorical exchange capacity. The effect of molecular sieve or exclusion due to cation volume may be responsible for such a limit.
Both Breck's and Townsend's arrangements of the isotherms indicate whether or not the cation in solution is preferrably exchanged by zeolite. However, they provide no information on the type of exchange sites or whether they have similar exchange energies. This information is necessary for a better comprehension of the mechanism of the ion-exchange phenomenon, i.e., whether only one or more than one site is involved in the exchange process. The Kielland plot, obtained by the Kielland quotient, may provide us with information regarding the exchange mechanism.
XAZ
XAS
XAZ
(d)
Figure 4: Arrangement of isotherms described by Townsend (1991): a. first type; b. second type; c. third type; d. fourth type.
The Kielland quotient (Kc) is defined as
,
where XAZ stands for the equivalent fraction of A, which is initially in solution in the zeolite; XBZ stands for the equivalent fraction of B, the cation originally in the solid phase that remained in the zeolite; asB stands for the molal activity of B in solution; asA stands for the molal activity of A in solution; ZA stands for the cation A charge; and ZB stands for the cation B charge. One can notice that for each isotherm point, i.e., for each concentration of the solution, there is only one corresponding equivalent fraction, XAZ, and therefore only one value of Kc. In the construction of isotherms, the maximum quantity of exchange seldom reaches 100% (XAZ=1). Barrer and Klinowski (1972-a) suggested that XAZ values should be normalized.
The values of Kc' (with XAZ') may be obtained in this way. The Kielland plots are merely plots of log Kc' versus XAZ'. Sigmoid isotherms of the second kind are characterized by plots which sometimes approach straight lines:
log Kc'= Co + 2C1XAZ',
where Co and C1 stand for coefficients. C1 is negative in sign. Sigmoid isotherms of the third kind give Kielland plots with a positive C1 if the linear function is obtained.
When the Kielland plots are linear, the zeolite is assumed to have only one kind of exchange site, but when AZA+ ions enter, an extra energy term (W) arises if pairs of AZA+ happen to occupy adjacent sites. This term is taken to be additive pairwise; and no such term arises if 2 BZB+ or AZA+ and BZB+ occupy adjacent sites. This phenomenon explains why the exchange is never complete (XAZ <1) (Barrer, 1974). Negative values of C1 correspond to positive values of W, so the extra energy term is endothermic and AZA+ ions tend to avoid one an other. Positive values of C1 correspond to extra exothermic pairing energy and the AZA+ ions tend to cluster.
MATERIALS AND METHODS
Zeolite Pretreatment
Pretreated samples with dp > 80 mesh and dp < 200 mesh were used in a 2N NaCl solution. Portions of 10g of zeolite with dp > 80 mesh were shaken in a mechanical shaker with five bed volumes of solution for one hour. Each portion was filtered in a Büchner funnel and washed with 50 ml of solution and 50 ml of deionized water. Afterwards, the samples were also dried at 373K for one hour. The procedure was repeated four times and the zeolite samples were washed again with five bed volumes of deionized water and dried. The dp < 200 mesh zeolite samples followed the described procedure, but were shaken in a magnetic shaker.
Ion Exchange Isotherm Construction
The procedure carried out was described by Barros et al. (1995). Ion exchange isotherms were constructed using a constant mass of pretreated zeolite in such a manner that the percentage of dried mass was 0.5%. The pH of the solution was measured, without correction, at different isothermal temperatures. pH values under 2 are considered critical for the dissolution of the aluminum framework (Huang and Hao, 1989; Zamzow and Murphy, 1992) being therefore should be avoided. Erlenmeyer flasks were stirred continously for 8 days, since it was supposed that this time would be sufficient for the system to attain equilibrium. Three isotherms were obtained at 298K, 313K and 333K. The 313K isotherm was obtained using a mesh of dp < 200 and for the others, a mesh of dp < 80 was used. However, a comparison of them is possible because this parameter has little influence on the exchange behaviour.
The isothem data were calculated by
XAS = and XAZ =
,
where C stands for the final concentration of chromium (equivalents in solution after 8 days), Co stands for the initial chromium concentration (equivalents) and CEC stands for the cation exchange capacity (Al equivalents presented in the zeolite sample, corresponding to 0.5% of the dried mass).
Construction of Kielland Plots
The Kielland quotients were obtained according to the equation shown in the Introduction. XBZ was considered to be XBZ = 1- XAZ. ZB is the charge of the chromium ion, +3, and, supposing that only sodium ions are exchanged, ZA was considered to be +1. The experimental points of the isotherms were fitted to equations and the functions XAZ=f(XAS) calculated with the help of the software package Table Curve. In this way, the curve points were normalized and they were used to calculate Kc. Afterwards, Kielland plots were constructed for the three isotherms.
RESULTS AND DISCUSSION
All analyses of the elements presented here were made by atomic absorption spectrophotometry. The exchangeable cations analysed were Na, K and Ca, which are the most abundant ones in this kind of zeolite. The data presented for these elements in Table 1 are normalized and, therefore, indicate the relative importance of each cation, without expressing its real concentration.
The cation exchange capacity, i.e., the sum of all aluminum, in the framework and impurities, was estimated as 397 meq/100g of zeolite. It can be seen in Table 1 that the procedure for the pretreatment used was not enough for the zeolite to become homoionic. Nevertheless, one verifies a substantial increase in the concentration of sodium ions. Figures 5a, 5b and 5c present the ion-exchange isotherms experimentally obtained. The functions XAZ=f(XAS) obtained by the Table Curve are described in Table 2.
ZNT = natural zeolite, without pretreatment, ZT = pretreated zeolite
200 = dp < 200 mesh, 80 = dp > 80 mesh
80-1 = first sample, used for the construction of isotherm at 298K
80-2 = second sample, used for the construction of isotherm at 333K
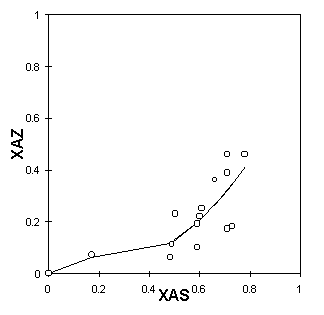
(a)
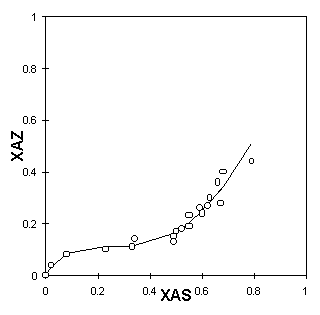
(b)
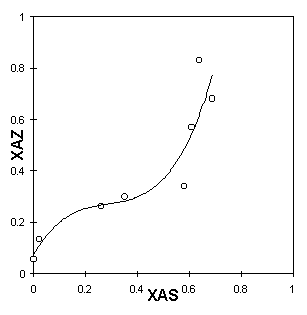
(c)
Figure 5: Ion exchanging isotherms in pretreated natural clinoptilolite: a - 298K, b - 313K, c- 333K
One observes that the isothermal behaviour is sigmoid, very similar to type "b," described previously. The sigmoid shape becomes even more characteristic as the temperature increases. Near the origin, the 298K isotherm has an almost imperceptible inflexion point. At higher temperatures, however, the inflexion point is more pronounced, making it possible to state the type of exchange behaviour.
Figures 6a, 6b and 6c show the experimental Kielland plots that appear as straight lines with positive values of C1, indicating that "W" is exothermic.
(a)
(b)
(c)
Figure 6: Kielland plots: a- 298K, b-313K, c-333K.
CONCLUSION
The pretreatment used was not able to assure the presence of only one kind of exchangeable cation in the zeolite. Nevertheless, there was a significant increase in the sodium concentration in the samples. The type "b" isotherms indicate that the natural clinoptilolite prefers the Cr+3 ion only when in contact with diluted solutions, as demonstrated by its downwards concavity. Since the inflexion point at 298K is almost imperceptible, one might mistake this isotherm for one of type "c" . Probably the Cr+3 has difficulty entering because of its quite large hydrated radius, close to micropore channel size. Observing the inflexion point, it can be concluded that these ions, even with a high charge, take on steric problems.
The mathematical treatment performed to obtain the Kielland plot improves the visualization of the straight lines. It indicates that the exchange sites have the same energy. Nevertheless, the mineral analysed has two kinds of zeolite (mordenite and clinoptilolite) besides the three main kinds of exchangeable cations; hence, it should display several exchange sites of different energies. As the Kielland plots are linear, we believe that only one kind of site acts in the exchange process with Cr+3.
The straight lines present positive values of C1, indicating that "W" is exothermic and that the Cr+3 ions tend to cluster. The degree of exchange increases as the temperature increases, because it apparently inhibits this tendency to cluster. It is observed that the higher the temperature, the more regular the distribution of the Cr+3 ions through the sites. Thus, the zeolite is able to shelter a greater quantity of these ions, elevating the inflexion point.
NOMENCLATURE
asA Molal activity of A in solution
asB Molal activity of B in solution
CEC Cation exchange capacity
dp Particle diameter
Kc Kielland quotient
Kc Normalized Kielland quotient
XAS Equivalent fraction of cation A in solution
XAZ Equivalent fraction of cation A in the zeolite
XAZ Normalized equivalent fraction of cation A in the zeolite
XAZmáx Maximum equivalent fraction of cation A in the zeolite
XBZ Equivalent fraction of cation B in the zeolite
ZB Cation B charge
ZA Cation A charge
W Extra energy therm
REFERENCES
Ackley, M.W.; Giese, R.F. and Yang, R.T., Clinoptilolite: Untapped Potencial for Kinetic Gas Separations, Zeolites, 12, 780-88, Sep-Oct (1992).
Alietti, A., Polymorphism and Crystal-Chemistry of Heulandites and Clinoptilolites, American Mineralogist, 57, 1448-62 (1972).
Barrer, R.M., Isomorphous Replacement by Ion Exchange: Some Equilibrium Aspects, Bull. Soc. fr. Minéral. Cristallogr., 97, 89-100 (1974).
Barrer, R.M. and Klinowski, J., Ion Exchange Involving Several Groups of Homogeneous Sites, J. Chem. Soc. Faraday Trans. I, 68, 73-87 (1972a).
Barrer, R.M. and Klinowski, J., Influence of Framework Charge Density on Ion exchange Properties of Zeolites, J. Chem. Soc. Faraday Trans. I, 68, 1956-63 (1972b).
Barrer, R.M. and Klinowski, J., Theory of Isomorphous Replacement in Aluminosilicates, Phil. Trans. R. Soc. Lond., 285, 637-80 (1977).
Barros, M.A.S.D.; Machado, N.R.C.F. and Sousa- Aguiar, E. F., Construção de Isoterma de Troca Iônica em Zeólitas Naturais, In. Encontro Regional de Catálise do Estado de São Paulo, 3, 1995. Campinas. Anais ... Campinas, 47-50 (1995).
Blanchard, G.; Maunaye, M. and Martin, G., Removal of Heavy Metals from Waters by Means of Natural Zeolites, Water Research, 18, 12, 1501-07 (1984).
Breck, D.W., Zeolite Molecular Sieves, Robert E. Krieger Publishing Company, Malabar, FL, USA (1984).
Czárán, E.; Mészáros, Á.; Domokos, E. and Papp, J., Separation of Ammonia from Wastewater Using Clinoptilolite as Ion Exchanger, Nuclear and Chemical Waste Management, 8, 107-13 (1988).
C'eranic', T.S., Lukic', T. M., Maximal Exchange Levels of Some Transition Metal Ions in Sodium Clinoptilolite, J. Serb. Soc., 9-10, 547-54 (1989).
Chen, S.H.; Chao, K.J. and Lee, T.Y., Lanthanium-NaY Zeolite Ion Exchange Thermodynamics and Thermochemistry, Ind. Eng. Chem. Res., 29, 2020-23 (1990).
Duffy, S.C. and Rees, L.V.C., Comparison of Experimental and Theoretical Rates of Ion Exchange, Journal of Chromatography, 102, 149-53 (1974).
Dyer, A.; Amini, S.; Enamy, H.; El-Nagar, H. A. and Anderson, M.W., Cation-exchange in Synthetic Zeolite L: the Exchange of Hydronium and Ammonium Ions by Alkali Metal and Alkaline Earth Cations, Zeolites, 13, 281-90, April-May (1993).
Franklin, K.R. and Townsend, R.P., Prediction of Multicomponent Ion-exchange Equilibria in Zeolites: A Comparision of Procedures, Zeolites, 8, 5, 367-75, September (1988).
Gaines, G.L.Jr. and Thomas, H.C., Adsorption Studies on Clay Minerals. II. A Formulation of the Thermodynamics of Exchange Adsorption, The J. Chem Phys., 21, 4, 714-18, April (1953).
Glueckauf, E., Activity Coefficients in Concentrated Solutions Containing Several Electrolytes," Nature, 163, 414-415 (1949).
Harjula, R.; Lehto, J.; Pothuis, J.H.; Dyer, A. and Townsend, R.P., Ion Exchange in Zeolites," J. Chem. Soc. Faraday Trans., 89, 11, 1877-82 (1993).
Huang, C.P. and Hao, O.J., Removal of Some Heavy Metals by Mordenite,Environmental Technology Letters, 10, 863-74 (1989).
Lee, T.Y.; Lu, T.S.; Chen, S.H. and Chao, K.J., Lanthanium-Na-Y Zeolite Ion Exchange. 2. Kinectics, Ind. Eng. Chem. Res., 29, 2024-27 (1990).
Loizidou, M.; Haralambous, K.J.; Loukatos, A. and Diimitrakopoulou, D., Natural Zeolites and Their Ion Exchange Behavior Towards Chromium, J. Environ., Sci. Health, A27, 7, 1759-63 (1992).
Maron, S.H. and Prutton, C.F., Fundamentos de Fisicoquimica, Ed. Limusa, México, 15 th Ed. (1984).
Nightngale, E.R.,Jr., Phenomenological Theory of Ion Solvation. Effective Radii of Hydrated Ions, The Journal of Phys. Chem., 63, 1381-87 (1959).
Ohlweiler, O.A., Química Inorgânica, vol. II, Ed. Edgard Blücher Ltda, São Paulo, SP (1973).
Pansini, M.; Colella, C. and Gennaro, M., Chromium Removal from Water by Ion Exchange Using Zeolite, Desalination, 83, 145-57 (1991).
Semmens, M. J. and Martin, W.P., The Influence of Pretreatment on the Capacity and Selectivity of Clinoptilolite for Metal Ions, Water Res., 22, 5, 537-42 (1988).
Sherry, H., The Ion - Exchange Properties of Zeolites. IV. Alkaline Earth Ion Exchange in Synthetic Zeolites Linde X and Y, The Journal of Physical Chemistry, 72, 12, 4086-94, November (1968).
Townsend, R.P., Ion Exchange in Zeolites, In Flanigen, E. M. and Janson, J.C., Studies in Surface Science and Catalysis, vol. 58: Introduction to Zeolite Science and Practice, chap. 10, Elsevier Science Publishers, 359-90, Amsterdam, Netherlands (1991).
Zamzow, M.J., Murphy, J.E., Removal of Metal Cations from Water Using Zeolites, Sep. Sci. and Technol., 27, 14,1969-84 (1992).
Publication Dates
-
Publication in this collection
09 Oct 1998 -
Date of issue
Sept 1997
History
-
Accepted
05 Aug 1997 -
Received
05 Mar 1997